Nitrogenous Base Definition
Several chemicals with a similar cyclic structure, each known as a nitrogenous base, play several important roles in biology. Not only is a nitrogenous base the building blocks for genetic information carrying molecules like DNA and RNA, but different forms of the nitrogenous base serve in various cellular roles from signal transduction to growing microtubules.
In DNA and RNA, a nitrogenous base forms a bond with a 5-sided carbon sugar molecule, which forms a “backbone” for the entire molecule. A nitrogenous base plus this sugar backbone is known as a nucleotide, and forms the building blocks of DNA and RNA.
Nitrogenous Base within Nucleic Acids
Purines and Pyrimidines
When talking about a nitrogenous base in the context of DNA or RNA, it is important to note that there are two base classes of nitrogenous base. Every nitrogenous base shares one feature: a six-sided ring with 4 carbon atoms and 2 nitrogen atoms. A purine has an additional 5-sided ring, created by 1 more carbon and 2 more nitrogen atoms. A pyrimidine nitrogenous base has only 1 six-sided ring. Each nitrogenous base has unique bonds, which makes it function in a unique way within the DNA or RNA. Each base can be seen in the image below.
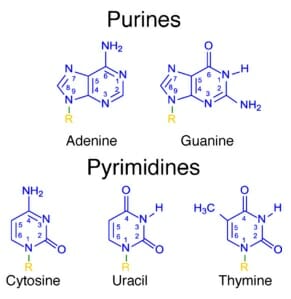
Purines and Pyrimidines
Deoxyribonucleic Acid (DNA)
The image below shows the structure of DNA. DNA has a “backbone” of deoxyribose, shown here as the colorless molecules with a 5’ and 3’ end. These numbers refer to the exposed carbons in the sugar chain, which gives DNA its directionality and readability. This allows various proteins to read and process the DNA efficiently.
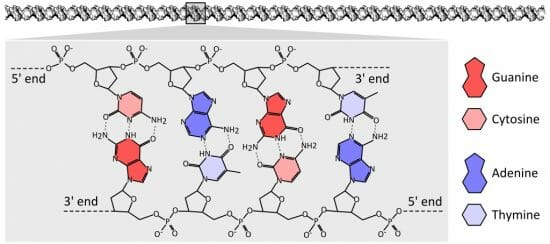
DNA chemical structure
Each colored molecule represents a nitrogenous base. Note how each nitrogenous base pairs with the nitrogenous base across from it. This is called base pairing, and is an important part of DNA replication, repair, and maintenance. Seen here in a proper configuration, each pyrimidine pairs with a purine, allowing several hydrogen bonds to be formed. These bonds, the dashed lines in the image above, hold the DNA in a regular spiraling shape, as well as protect the DNA from having a nitrogenous base accidentally break off.
Enzymes which repair and maintain the DNA can “sense” malformations caused by a lack of hydrogen bonding. If, for example, two purines tried to pair they would not be able to form hydrogen bonds. A repair enzyme would find a “bulge” or irregularity in the DNA. Certain enzymes can then slice out and replace the incorrect base.
Ribonucleic Acid (RNA)
There are two noticeable differences between RNA and DNA. The first is in the name itself. Where DNA is built on deoxyribose, RNA is built on ribose. The only difference between ribose and deoxyribose is an oxygen atom.
The second difference between DNA and RNA is that RNA uses a slightly different set of nitrogenous bases. Seen in the image below, an RNA molecule substitutes uracil for thymine. The reasons for this are not fully understood, although RNA is generally a shorter lived molecule. Further, RNA often exists as a single-strand, rather than a double-strand with hydrogen bonds. This is not always the case, as seen in double-stranded RNA viruses, but RNA is typically single stranded in most animals.
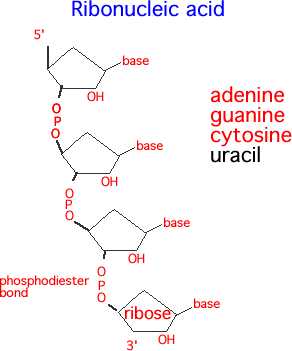
RNA structure
Regardless of whether the nucleic acid is DNA or RNA, the basic formula is the same. Take a nitrogenous base, add on a 5-carbon sugar with a phosphorous group, and bind together. The bonds formed between the phosphorous group and the oxygen of the next 5-carbon ring are called a phosphodiester bond, and form the backbone of both RNA and DNA.
How a Nitrogenous Base Carries Genetic Information
Each nitrogenous base carries little information itself. Rather, each nitrogenous base is read as a unit, with two other bases. These three-base information packets are called codons. Each codon specifies a certain amino acid. Put together in proper order and folded into shape, a chain of amino acids creates a protein. These proteins then carry out the functions of life, including everything from growth to reproduction.
It takes around 3,000,000,000 base pairs to create a functioning human. This means that there are around 6,000,000,000 individual bases in each cell of your body. While this may seem like an enormous amount, your body is constantly processing and replicating your DNA. This is probably the main and most important function of a nitrogenous base for any organism.
Nitrogenous Bases in Other Cell Functions
Energy Transfer
Genetic information storing is not the only task of a nitrogenous base. Many are used in the transfer of energy between food molecules like glucose and the energy needs of proteins within the cell. The most recognized of these molecules is adenine triphosphate, more commonly known as ATP. While biology textbooks often refer to this molecule as the cell’s universal energy transfer molecule, it is important to note that it is based on adenine, the nitrogenous base.
While ATP is widely recognized in a number of cellular reactions, it is not the only nitrogenous base that serves in cellular energy transfer. Another molecule, guanine triphosphate (GTP), is used in a number of cellular functions. GTP opens protein channels, aids in the formation of microtubules, and even energizes the import of important proteins into the mitochondria. This in turn helps produce more ATP via aerobic respiration, which powers the cell’s growth.
Cell Signaling
A nitrogenous base can also serve important roles in cell signaling, a process known as signal transduction. The general scheme involves a number of chemical messengers acting on various proteins within a cell to send a signal. A pancreas cell may measure the blood glucose, transduce a signal to release insulin, and disperse the insulin into the blood stream. This process is integrated and coordinated by a number of factors involving a nitrogenous base.
ATP and cyclic adenine monophosphate play important roles in intracellular signaling such as this. Their ratio drives various chemical reactions to different equilibrium points, which in effect drives the activies of the cell. GTP is implicated in a number of pathways from growth and metabolism to signaled cell death (apoptosis).
Quiz
1. How much information does a nitrogenous base carry?
A. One-third of one amino acid
B. None
C. One amino acid
2. Which of the following is NOT a nitrogenous base?
A. Adenine
B. Thymine
C. Ribose
3. A scientist is creating synthetic DNA in a laboratory. At first, he decides to use only two synthetic nitrogenous base molecules. Both of them resemble purine molecules. Which of the following problems will the scientist experiment?
A. Nothing, it should work fine.
B. The DNA will not be double stranded.
C. The DNA will twist too much.
References
- Hartwell, L. H., Hood, L., Goldberg, M. L., Reynolds, A. E., & Silver, L. M. (2011). Genetics: From Genes to Genomes. Boston: McGraw Hill.
- Lodish, H., Berk, A., Kaiser, C. A., Krieger, M., Scott, M. P., Bretscher, A., . . . Matsudaira, P. (2008). Molecular Cell Biology (6th ed.). New York: W.H. Freeman and Company.
- Nelson, D. L., & Cox, M. M. (2008). Principles of Biochemistry. New York: W.H. Freeman and Company.
Nitrogenous Base